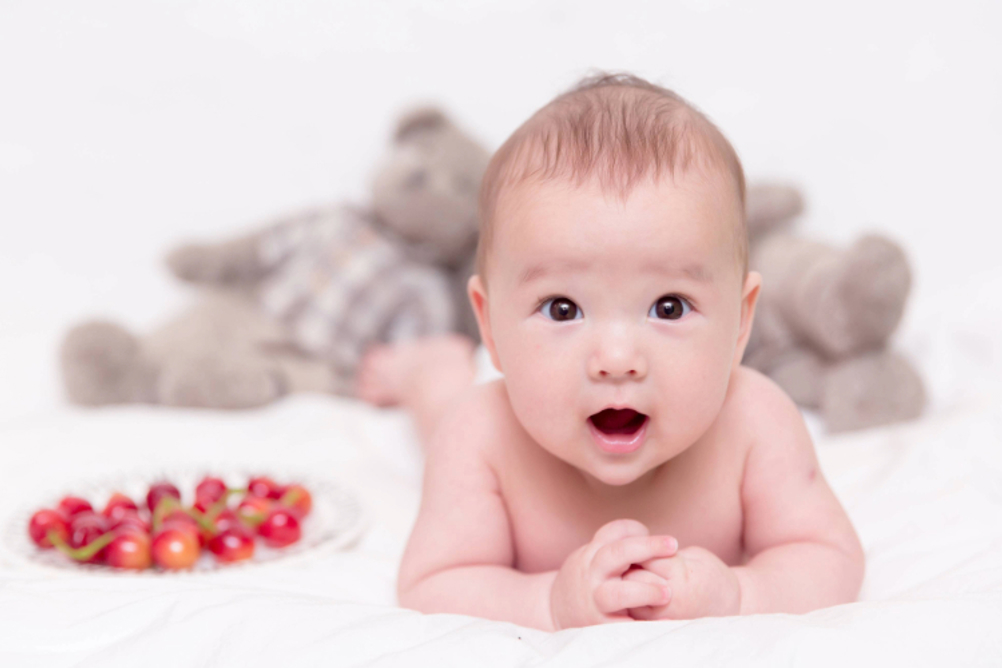
While medical advances occur on a wide range of clinical frontiers, perhaps the neonatal unit has witnessed some of the most significant degrees of progress in an area of increasing challenge. It is a relevant reflection that the neonatal intensive care unit is barely 50 years old in the United Kingdom.
Discovering retinopathy of prematurity
The increasing survival of babies of earlier gestation presents challenges relating to the development of the retina, where the migration forward of its frontier of active retinal cell growth is not complete until the 40th week of gestation. The condition of retrolental fibroplasia (RLF), first described by Terry in 1942,1 is now recognised as an advanced presentation of retinopathy of prematurity (ROP).2 Terry was a professor of ophthalmology at the Massachusetts Eye and Ear Infirmary in Boston. Such initial observations were subsequently linked to the more frequent delivery of supplementary oxygen to neonates, using incubators of more developed design.
In his observations, Terry was acting as the perceptive ophthalmologist and identified that the level of presentations of RLF was essentially a new phenomenon. Not being aware, however, of the increasing administration of oxygen, Terry was not able to initially identify this change in clinical practice for the increased observation of such cases.
A subsequent series of animal experiments identified the link between high oxygen levels post birth and RLF.3 This led to the restriction of high levels of unregulated supplemental oxygen, where subsequently in the mid-1950s this was found to have reduced the level of RLF but have increased the level of mortality and morbidity.
A cautionary tale
It is considered that as many as 10,000 babies suffered irreversible vision loss on account of administration of excess oxygen in the approximate period of 1942 to 1954. There is, however, remarkably little reference to such outcomes in the wider clinical literature. The lack of clinical foresight in preventing this occurrence is described by Jacobson and Feinstein4 in the context of a cautionary tale with the benefit of hindsight.
One significant factor was the assumption that elevated levels of oxygen were entirely beneficial and another factor was that of poorly constructed clinical studies to determine the cause of increased presentations of RLF.
Across a range of clinical investigations of the causation of RLF in this period between 1942 and 1954, various factors including the level of illumination, iron therapy, vitamin E levels and also oxygen administration were actively investigated.
In the period of such studies, however, there was no appropriate technology to continuously monitor the level of oxygen saturation in neonates, and the levels of inspired oxygen concentration were not always recorded. In addition, systems for administration of oxygen did not always deliver effectively. Part of the problem was that the condition of RLF could present in babies with no supplementary oxygen exposure, indicating that such exposure was not a necessary or sufficient cause of RLF, though in time its role would be identified as a contributory one.
One key element, which hampered research into the origins of RLF, was that across various studies undertaken, there was no formal agreed diagnostic criteria that could confirm the presentation of RLF. Linked with this was also the lack of consensus as to when eye examination should be undertaken.
Studies, for example, undertaken by Reese et al,5 where the majority of observations were made two months after birth, were used to indicate that RLF was probably prenatal in origin. It is a feature of modern clinical studies that diagnostic criteria are closely defined with also appropriate identification of relevant timelines of clinical observation.
One hypothesis investigated by Terry was that the level of illumination to which neonates were exposed could be a contributing factor;6 this prompted various studies where neonates were blindfolded to various extents after birth and where it was eventually identified7 that there was no link with light exposure.
Another false trail was that of association of RLF with haemangiomas, as superficial vascular features and where the issue was subsequently identified as ‘detection bias’, where an effective control group had not been established to identify a base line for comparison.
Improving validation of oxygen status
The first advance in instrumentation to monitor levels of neonatal oxygen saturation was the development of the transcutaneous oxygen sensor, where a measurement was made of perfused surface tissue oxygen levels as measured by a Clark electrode unit to measure TcPO2 levels. Dual electrodes were also developed to measure also TcPCO2 levels using the Severinghaus type electrode.
A key element of such an electrode was a gas permeable membrane. It was necessary, however, to heat the tissue to around 44°C to establish measurement accuracy and reduce the time to reach gas equilibrium. Due to the thermal stress on the skin surface, the electrode required to be repositioned and recalibrated every few hours.
A subsequent development was that of the pulse oximeter which determined the level of oxygen bound to haemoglobin in the neonate; this factor essentially measured the level of oxygen available for gas exchange but not the actual partial pressure of oxygen present in the tissue. While introduced across all acute disciplines, the technology was especially appreciated in neonatology, where the requirement for frequent repositioning and calibration of electrode systems was no longer required.
Appropriate care is required in placement of SpO2 probes on the neonate and where devices are often attached over the neonate’s foot. Figure 1 indicates a typical Masimo pulse oximeter probe intended for neonatal use. The basic function of the SpO2 probe is the measurement of light transmission at two separate wavelengths. The detection algorithm is based on the fact that deoxygenated haemoglobin absorbs more light in red wavelengths around 650nm while oxygenated haemoglobin absorbs more light in the infrared wavelengths around 900nm.
Figure 1: Masimo neonatal spO2 probe: Small dots in left aperture are the emitting LEDs – about 200 microns in diameter (scale in mm)
In routine use, the pulse oximeter has essentially entirely replaced the transcutaneous sensing technology in neonatology and SpO2 probes are used without requirement for individual calibration. The accuracy of each SpO2 probe product relates essentially to the control of the spectral output characteristics of the red and infrared LEDs, as characterised by their peak wavelength.
While the checking of the wavelength accuracy of individual probes is not advocated by device manufacturers, a UK company has developed a product that undertakes such a measurement and for a set of identified SpO2 probes. The device can make an assessment of the relative accuracy of a specific SpO2 probe by measuring the spectra of the separate LEDs and relating this to the reference spectral distribution of the LEDs from which the definitive SpO2 calibration was originally derived.
The oxygen dissociation curve
An important element of appreciation of the role of measurement technology to determine SpO2 and pO2 is indicated in figure 2, where for blood with essentially neonatal haemoglobin, higher saturation levels of SpO2 are achieved for a given level of pO2; this gives neonatal haemoglobin a higher oxygen carrying efficiency.
As higher levels of SpO2 are achieved, the uncertainty in corresponding pO2 levels increases, where fluctuations around 95% values of SpO2 values can correspond to significant changes in pO2 values. Where neonates received packed adult red blood cells to augment levels of haemoglobin, this can also alter the achieved levels of SpO2.
Figure 2: Typical oxygen dissociation curve for ‘neonatal’ blood and ‘adult’ blood
The Boost II Trials
Studies8 had suggested that a target SpO2 level below 90% could reduce the incidence of ROP but without adversely affecting mortality or morbidity. In an effort to more clearly identify clinical outcomes with varying target levels of SpO2, parallel trials (Boost II) were conducted in the UK and Australia, where the primary outcome was the level of death or major disability at 18 to 24 months, with age corrected for prematurity for neonates born before 28 weeks gestation.
Subjects were randomly assigned to one set of pulse oximeters that would read up to 3% higher than the actual value, for example one that read 90% would in reality be 87%. The other set would in fact reflect a higher value than indicated, where, for example, a value of 90% would in fact be as high as 93%. The set target SpO2 reading of both sets of pulse oximeters was in the range 88% to 92%.
Figure 3 indicates the relative readings of the two sets of devices relative to ‘true’ levels of SpO2. Recruitment into the trial was halted when it was identified that there was increased mortality at 36 weeks corrected gestational age for the lower-target SpO2 group. Subsequent analysis also indicated significantly higher rates of death or disability at two years for the lower-target groups for both the trial in the UK and Australia.
It was identified that more subjects were treated for ROP in the higher-target group, though there was no significant difference in the two groups in relation to blindness. This confirmed that there are unacceptable clinical risks in lowering SpO2 levels to attempt to reduce the occurrence of ROP. A detailed account of the Boost II study9 provides also an indication of the significant complexity of structuring such studies, undertaking their analysis and relating findings to clinical practice.
Figure 3: Correction algorithm of lower-reading and higher-reading spO2 monitors
Developments in oxygen monitoring
There have, however, been developments in basic oxygen sensor technology which could introduce increased accuracy in measurement of oxygen gas delivery systems. Using a process of optical measurement based on the response of a luminescent sensor dye to the concentration of oxygen, various companies10 have developed sensors with significantly superior properties to that of the electro-galvanic oxygen device.
The stability, reliability and shelf life of such conventional devices present challenges to manufacturers and service agencies of medical equipment. In operation the luminescent sensor dye is illuminated by an LED until equilibrium of fluorescence is achieved. The LED is then switched off and the decay of fluorescence monitored and where increasing oxygen concentration will increase the rate of decay of fluorescence. A summary of characteristics of a cell type developed by PyroScience is indicated in table 1 (right).
Figure 4 indicates the excitation and fluorescence decay episodes where the output fluorescence decay signal is progressively ‘quenched’ by higher levels of oxygen. A similar principle can also be implemented for CO2 detection.
A PyroScience sensor using this technology is currently on board the Moxie module in Nasa’s Perseverence Rover on Mars,11 which monitors the conversion of CO2 from the Martian atmosphere to oxygen. The technology also allows measurement of oxygen concentration at the small scale using fibre optic probes.12 This technology, therefore, may also be relevant to monitoring of levels of oxygen in studies of contact lens use.
This branch of technology also provides the potential for the use of new sensing modality for transcutaneous oxygen probes13, 14 that would likely not require individual calibration. While this technology is unlikely to challenge the dominance and convenience of pulse oximeter probes, the additional measurement of such transcutaneous oxygen probes could assist in the management of administration of supplementary oxygen in both the delivery room and the neonatal unit. A recent review article by Bernasconi et al, however, provides a wide ranging analysis of emerging technologies for transcutaneous probes for both pO2 and pCO2.15.
There has been also interest in contact-less technologies for monitoring of oxygen saturation. Kong et al describes a system where a subject is illuminated under ambient light and two video cameras with narrow band filters at 520nm and 660nm monitor a region of the subject’s face.16
Extraction of the pulsatile profile of the video signals provides data for derivation of the SpO2 parameter derived from the reflected light, while a conventional signal is captured using a standard SpO2 finger probe. While good agreement is claimed between the two measurement techniques, the image analysis measurements were undertaken on non-moving subjects and where analysis of moving surfaces in real time would be a significant issue to resolve. An attraction of this technique is the potential to monitor several areas of the clinical subject, ‘in parallel’, with the utilisation of significant levels of computer processing power.
Figure 4: Excitation and fluorescence decay episodes of activated device
In the delivery room
While there has been some degree of clarification of the post birth maintenance levels of oxygen saturation of the neonate in the neonatal unit, the time line of the process of birth presents challenges in the establishment of stable levels of oxygen saturation, where the conditions of hypoxia (oxygen deficiency) and hyperoxia (high levels of oxygen) present separate challenges.
In summary, the lessons learned from history for post-birth neonates have not been translated into delivery room practice. Thus it was the case that the International Liaison Committee on Resuscitation (ILCOR)17 from 1992 till 2005 essentially recommended 100% oxygen for delivery of infants with a degree of difficulty at birth. There has generally however, been a trend to avoid using high oxygen concentrations as the hazard of oxygen free radicals and oxidative stress coupled with low anti-oxidant capacity in preterm infants became increasingly apparent.18
In 2015, ILCOR recommended that neonatal resuscitation be initiated with concentrations of oxygen in the range 21% to 30% with avoidance of concentrations in the range 65% to 100%. The challenge is one to allow the SpO2 levels to rise appropriately without generating damaging free radicals from high levels of oxygen and also exposing the neonate to damaging effect of the lack of oxygen. One guidance figure from ILCOR is to achieve 80% of target SpO2 within five minutes of birth.
In the timeline of the delivery room, every second literally counts in ensuring an appropriate SpO2 recovery timeline. Observations of researchers, however, indicate that a critical element is the level of experience and also the availability of attending staff in, for example, achieving prompt SpO2 monitoring, setting of prescribed levels of oxygen through gas mixing valves and achieving target profiles of SpO2 levels. Oei et al provide a reality check from observations on how well delivery room targets of rising SpO2 levels are actually achieved.19
Figure 5 indicates a widely used set of target profiles of SpO2 levels as a range of values to achieve. Using the graph of figure 2, the corresponding ranges of pO2 are also indicated. This identifies that the higher SpO2 value at 10 minutes has a relatively high level of uncertainty in pO2 values, on account of the profile of the oxygen dissociation curve as indicated in figure 2 for neonates. This is where a more exact measurement of pO2 values would be relevant to identify conditions of hyperoxia.
Figure 5: Post-birth scenario of target spO2 levels and implied pO2 levels from oxygen dissociation curve for neonatal blood
Summary
The description of the resolution of the link between supplementary oxygen and the development of ROP is cautionary to the extent that there are many issues within ophthalmology and vision science where there remain unresolved causal relationships.
Developments in oxygen sensing, using emerging optical techniques, have the potential to improve both oxygen delivery techniques and the measurement of perfusion of oxygen in tissue to both optimise neonate survival and reduce the incidence of ROP.
- Dr Douglas Clarkson is a research fellow at UHCW NHS Trust in Coventry with a wide interest in optical technologies applied to diagnostic specialties and modes of treatment.
References
- Terry TL. Extreme Prematurity and Fibroblastic Overgrowth of Persistent Vascular Sheath Behind Each Crystalline Lens: I. Preliminary report. Am J Ophthalmol. 142; 25; 203-4
- Loganathan PK, Nair V, Lal MK, Retinopathy of prematurity: overview of new global problem, Paediatrics and Child Health, 2022, 32(9), 311-323
- Ashton N, Ward B, Serpell G. Role of oxygen in the genesis of retrolental fibroplasia; a preliminary report. Br J Ophthalmol. 1953 Sep;37(9):513-20
- Jacobson RM, Feinstein AR. Oxygen as a cause of blindness in premature infants: “autopsy” of a decade of errors in clinical epidemiologic research. J Clin Epidemiol. 1992 Nov;45(11):1265-87
- Reese Ab, Payne F. Persistence and hyperplasia of the primary vitreous (tunica vasulosa lentis or retrolental fibroplasia). Am J Ophthalmol. 1946 Jan;29:1-24
- Terry Tl. Ocular Maldevelopment In Extremely Premature Infants: Retrolental Fibroplasia: Vi. General Consideration. JAMA. 1945;128(8):582–585
- Locke JC, Reese Ab. Retrolental fibroplasia; the negative role of light, mydriatrics, and the ophthalmoscopic examination in its etiology. AMA Arch Ophthalmol. 1952 Jul;48(1):44-7
- Tin W, Milligan DW, Pennefather P, Hey E. Pulse oximetry, severe retinopathy, and outcome at one year in babies of less than 28 weeks gestation. Arch Dis Child Fetal Neonatal Ed. 2001 Mar;84(2):F106-10.
- BOOST-II Australia and United Kingdom Collaborative Groups; Tarnow-Mordi W, Stenson B, Kirby A, Juszczak E, Donoghoe M, Deshpande S et al, Outcomes of Two Trials of Oxygen-Saturation Targets in Preterm Infants. N Engl J Med. 2016 Feb 25;374(8):749-60
- Wolfbeis OS. Luminescent sensing and imaging of oxygen: fierce competition to the Clark electrode. Bioessays. 2015 Aug;37(8):921-8
- Jeffrey A. Hoffman et al,Mars Oxygen ISRU Experiment (MOXIE) ¬ Preparing for human Mars exploration. Sci. Adv.8,eabp8636(2022).DOI:10.1126/sciadv.abp8636
- Werner J, Belz M, Klein K, Sun T, Grattan KTV, Evaluation and optimization of the performance characteristics of fast response fiber optic oxygen gas probes, Sensors and Actuators A: Physical, 365,2024, 114933
- Vakhter V, Kahraman B, Bu G, Foroozan F, Guler U, A Prototype Wearable Device for Noninvasive Monitoring of Transcutaneous Oxygen, IEEE Transactions on Biomedical Circuits and Systems, 2023,17(2), 323-335
- Costanzo I, Sen D, Guler U, An Integrated Readout Circuit for a Transcutaneous Oxygen Sensing Wearable Device, 2020 IEEE Custom Integrated Circuits Conference (CICC), Boston, MA, USA, 2020, 1-4
- Bernasconi S, Angelucci A, De Cesari A, Masotti A, Pandocchi M, Vacca F et al, Technologies for Transcutaneous Oxygen and Carbon Dioxide Monitoring. Diagnostics (Basel). 2024 Apr 9;14(8):785.
- Kong L, Zhao Y, Dong L, Jian Y, Jin X, Li B, Feng Y, Liu M, Liu X, Wu H. Non-contact detection of oxygen saturation based on visible light imaging device using ambient light. Opt Express. 2013 Jul 29;21(15):17464-71
- Niermeyer S, Kattwinkel J, Van Reempts P, Nadkarni V, Phillips B, Zideman D et al, International Guidelines for Neonatal Resuscitation: An excerpt from the Guidelines 2000 for Cardiopulmonary Resuscitation and Emergency Cardiovascular Care: International Consensus on Science. Contributors and Reviewers for the Neonatal Resuscitation Guidelines. Pediatrics. 2000 Sep;106(3):E29.
- Negi R, Pande D, Kumar A, Khanna RS, Khanna HD. Evaluation of biomarkers of oxidative stress and antioxidant capacity in the cord blood of preterm low birth weight neonates. J Matern Fetal Neonatal Med. 2012 Aug;25(8):1338-41
- Oei JL, Finer NN, Saugstad OD, Wright IM, Rabi Y, Tarnow-Mordi W, Rich W, Kapadia V, Rook D, Smyth JP, Lui K, Vento M. Outcomes of oxygen saturation targeting during delivery room stabilisation of preterm infants. Arch Dis Child Fetal Neonatal Ed. 2018 Sep;103(5):F446-F454